"Learning corner"
Electron microscopes
All properties of the objects that surround us in our daily life are caused by placement of different atoms in those materials. Thus, by observing the inner of a material, atom by atom, we should be able to predict the exact mechanical, electrical, magnetic or chemical property of a material. The microscope that can obtain information about the placement of atoms inside a material is the transmission electron microscope, TEM. Therefore, it is the TEM that gets closest the above vision, i.e. determining the materials properties from the knowledge of placement and type of atom in their inside.
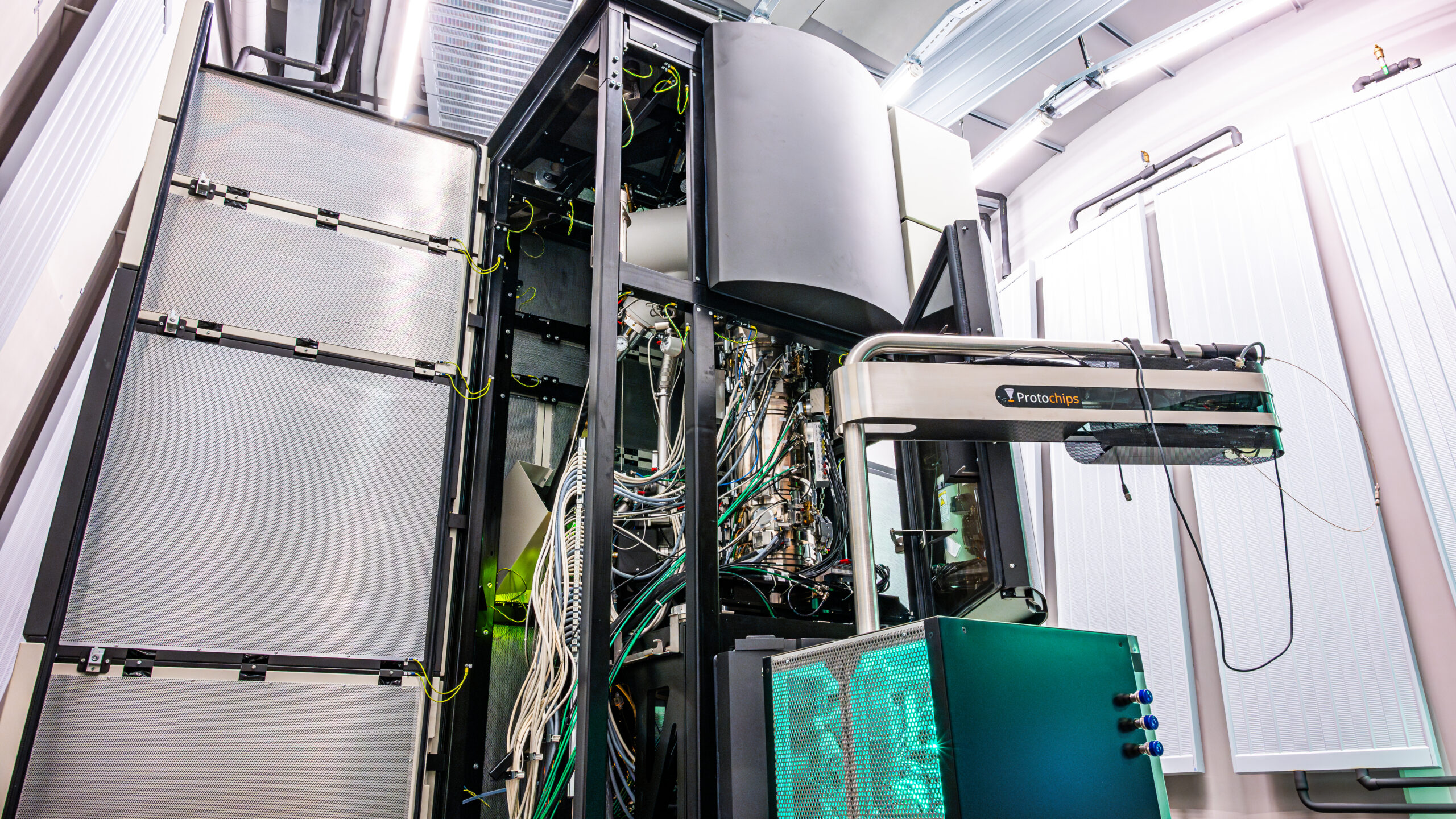
As you can guess, one of the difficulties in this task is that there is a huge number of atoms in a material. Since we cannot analyse every single atom in a material (very very time consuming!), it necessary, to choose certain places in a material that are believed to be crucial to understand the functioning of it.
A second difficulty consists in preparing a sample such that it is suitable for this analysis method, transmission electron microscopy. Since we want to look into the inside of a material by using electrons, the electrons must enter the material where they will interact with its atoms. After this interaction, the electrons will change their character, which means that either they will change their direction or their energy. Aha, let’s see how we could then detect those electrons. The easiest way to detect those electrons is to make our sample so thin, that the electrons, after entering the sample, could leave the sample easily on the opposite side. And this is actually how we do in transmission electron microscopy. What seems so straight forward, needs though a bit of attention, because for our very fast electrons to be able to transmit the sample, the latter must be very thin, i.e. typically 1/10.000 part of a millimeter thin.
Once that we have prepared such a nice sample, we will put it in the TEM. This instrument has been developed over the past 80 years with exciting progress in every single year during this development time. How does a modern TEM work?
The beam optics in a TEM
If you have looked into a light microscope, you might remember that this microscope works in the following way. Light is emitted from a point like source, typically at the bottom of the microscope and then it is converged onto the sample by a condenser lens. Many of you will have looked at biological samples that where fixed on a glass slide. When the light enters the sample, it will be so scattered, which means that a part of the light will change its direction. Often, the biological samples are transparent to light which means that the light enters the sample on the lower border and exits it at its upper surface. Using the objective lens, the scattered light is captured and an image is created in the image plane of this lens. Since the magnification of this objective lens is rather limited, this image is very small and we need an ocular to see the image of the sample with our bare eye or with a digital camera.
The transmission electron microscope has the same optical beam diagram as described above, but with a few differences: We use electrons instead of light and the electron beam in a typical TEM moves from up to down as compared to the light microscope where it moves from down to up. Using electrons sounds strange, doesn’t it? Electrons are a particle, light is a wave. And, in fact, physics says that for most observations in an optical microscope, you need a wave. The nature of the electron though is a tick more complicated. Though it is a particle, quantum theory and related experiments, about 100 years ago, have found that the electron has also wave nature. And due to this wave nature, we can use lenses and we can understand the optical properties of a TEM in a similar way as we understand the optical properties of a light microscope. Though, didn’t we learn above that for a material to be transmitted by the fast electrons in the TEM, it has to be very thin? Well, the glass lens in a light microscope are not, they are millimeters or centimeters thick. Thus, the electrons would simply get stuck in the glass, if we used such lenses in the TEM. Therefore, particle lenses had to be developed to make a TEM work. Theses lenses deviate or refract the electron beam by magnetic or electrical fields and are the heart of our TEM. It has turned out that we can use a magnetic objective lens in the TEM and this lens will have an image plane as the similar lens in the light microscope.
You have seen that building a TEM and also to preparing suitable samples is quite an effort and you might have asked yourself: what for? The answer is straightforward: RESOLUTION.
Imaging and Spectroscopy in the TEM
Scientists have found out that the resolution of an optical microscope, whatever will be the nature of the wave moving in it, is directly proportional to the wavelength of it. Visible light has a wavelength that is about half a micrometer (1 micrometer = 1/1.000 millimeter) and consequently, the resolution of the light optical microscope is of the order of half a micrometer. The electrons in the TEM have a typical wavelength of about 20 picometers (1 picometer = 1/1.000.000 micrometer) and a typical resolution of a TEM is in the range of 1 Å (1Å = 1/10.000 micrometer). This fantastic resolution makes it possible to see where the atoms are placed in our material and to know which type atom is on the place of analysis!
To obtain an image, we use the exact beam path of electrons in our TEM as was described for the light microscope above. Of course, we need to employ a camera that is sensitive to electrons in our TEM. And then we will see atomic resolution images of our sample.
But this still does not answer us the question, which is the type of atom that we observe at this place. From images we can obtain some information giving us a hint on which atom we have at one place by observing with which intensity the atom appears in the image. This is certainly very useful information.
Still, if we want to unambivalently determine if we have, for example, and iron atom or a vanadium atom at one place in our sample, we need so-called spectroscopy techniques. These techniques give us a fingerprint of the atom that is under the electron beam. This fingerprint appears as a peak or an edge in the spectrum that is acquired using these techniques. So, from where do these techniques get the fingerprint of an atom? To understand this, we have to move one step further into the atom being composed of the positive nucleus and the electronic shells or more precisely, electronic orbitals. Every orbital has a very well-defined energy. Therefore, if we excite an electron from one orbital to an orbital with a higher energy (or into the vacuum), the electron will have acquired an amount of energy corresponding to the difference in energy between these two orbitals. This energy difference is characteristic for the orbitals of every atom in the periodic system. The amount of energy difference between two orbitals can be either measured through characteristic X-rays emitted from an atom after the primary excitation process or by determining the energy loss that the primary electrons experienced when the electrons in the atom were excited. In the first case, the technique is called energy dispersive X-ray spectroscopy, in the second electron energy loss spectroscopy.